Structural steels (TMCP)
Structural steels represent a large group of products ranging from fairly simple to highly sophisticated steels. Typical applications are found in infrastructural constructions, pressure vessels, machines, construction equipment, hoisting devices, mining & mineral processing equipment, offshore platforms, ships, or containers. Structural steels are delivered in various shapes such as strip, heavy plate, hollows, sections, beams, angles, and bars. Structural steels are delivered in as-hot rolled condition for the less demanding applications. However, in highly stressed steel structures, there is a strong interest in reducing the dead weight without losing load bearing capacity. The required high strength steels come in either normalized, thermomechanical rolled or heat-treated condition. The yield strength of high strength structural steels spans from 355 to nowadays 1300 MPa. Molybdenum is usually considered as an alloy option for strength levels of 500 MPa and above. According to industry standard EN 10025-4, molybdenum can be used to a maximum of 0.2% in in thermomechanical rolled steels. Industry standard EN 10025-6 allows a maximum molybdenum addition of 0.7% in all high strength structural steels to be treated by quenching & tempering.
Hot strip products can be rolled to maximum gages of 25 mm (1 inch). Thus, strip steels are most suitable for lighter construction purposes. Important applications are found in the manufacturing of heavy commercial vehicle, mobile cranes, hoisting equipment, and other industrial transportation equipment.
Heavy plates cover gages and lateral dimensions that cannot be met by strip mills. Therefore, they are preferred for the construction of infrastructure systems, offshore equipment, vessels, tanks, and heavy machinery components amongst many other applications.
The use of higher strength steel for such applications construction is often motivated by reducing the plate gage and thus the total weight while maintaining the load bearing capacity. The weight reduction of constructions brings about many advantages, such as lower material consumption, less welding work, as well as reduced transport and hoisting efforts. These advantages can often overcompensate the increased material cost per ton of the higher strength steel. Molybdenum is an increasingly important alloying element in structural steels with yield strength above 500 MPa.

Weight savings potential with increasing yield strength
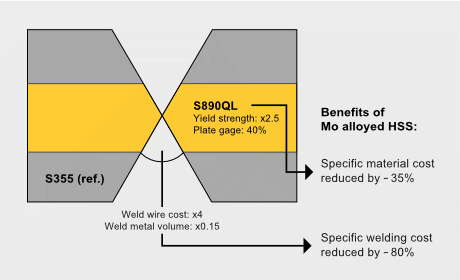
Reduction of welding efforts by increasing material strength and reducing gage
TMCP rolled structural steel
For smaller gages and up to strength levels up to 700 MPa TMCP rolling followed by accelerated cooling is the preferred approach for producing structural steels, either as strip or plate. The metallurgical principle of grain refinement, induced by microalloying (Nb, Ti) and additionally supported by molybdenum provides the basis for strength. Additional strength contribution is gained by accelerated cooling after finish rolling. The delay of phase transformation leads to even finer grain size and microstructure becomes increasingly bainitic. Molybdenum alloying supports the transformation delay effect by its metallurgical functionality which is particularly effective in combination with accelerated cooling. The metallurgical and processing approaches for TMCP treated structural steels is actually identical to those used for producing line pipe steels.
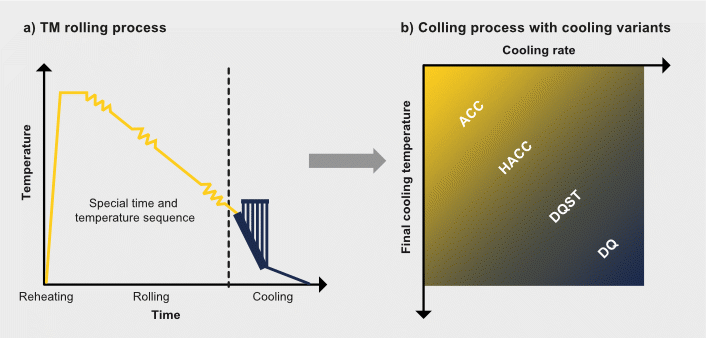
TMCP rolling and various cooling strategies after finish rolling
Direct quenched (DQ) structural steel
A more recent approach for economically producing structural steels with exceptionally high strength (YS ≧800 MPa) is the so-called direct quenching (DQ) process. These steel grades rely on a (tempered) martensitic microstructure. Immediately after the rolling process with the microstructure still being fully austenitic intensive cooling with high rate and low cool-stop temperature can produce a martensitic transformation. This quenched steel can be optionally tempered by self-tempering or by a separate annealing treatment. DQ processing is applicable to strip and plate products. As compared to classic reheat quenching (RQ) DQ saves energy and simplifies plant logistics. However, the possible applicable product gage for DQ processing is limited to approximately 30 mm. Molybdenum alloying, often applied in combination with B, Cr, and Ni, provides the necessary hardenability for the DQ process. Plate steels of 1000 MPa and strip steels with >1100 MPa were successfully produced using Mo additions of 0.5-0.7% (cross-references lit.). A major microstructural difference in comparison to conventional RQ steel having a normalized grain structure is that the DQ process is applied to a deformed austenite microstructure with a larger property anisotropy as a consequence. However, the final microstructure of DQ steels is usually finer-sized than that of RQ steels which may be beneficial to strength and toughness.
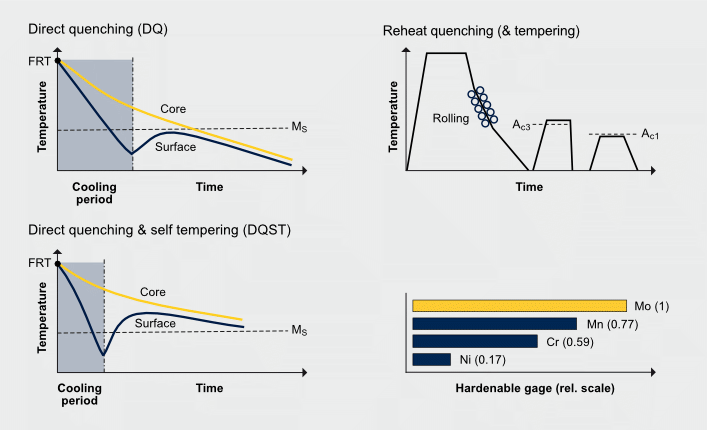
Production principles for producing martensitic or tempered martensitic steel (Ms: martensite-start temperature, Ac1,3:start and end of austenite transformation); relative effect of alloying elements on hardening depth.
Heat-treated (RQ) structural steel
High-strength, water-quenched & tempered structural steels are the material of choice to fulfil severe requirements in terms of strength and toughness. The respective steel grades range from a minimum yield strength of 550 up to 1100 MPa. A low carbon equivalent is mandatory if extensive welding is to be used as, e.g., in offshore engineering. The quenching operation starts with reheating to temperatures above Ac3, which have to be rather homogeneously distributed from the surface to the core of the plate. Subsequent quenching by pressurized water and in many cases supported by molybdenum’s hardenability effect guarantees a transformation of the microstructure into martensite or bainite across the thickness. The desired mechanical property combination in terms of strength and toughness is adjusted by controlled tempering at temperatures up to 700 °C. Here, molybdenum is very efficient in avoiding excessive loss of strength during tempering. The chemical compositions of the steel grades need to be adapted in detail to obtain the mechanical properties necessary for each specific application. High strength quenched & tempered structural steels usually comprise a carbon content of less than 0.3% and manganese contents of max. 2%. These steels typically contain certain additions of alloying elements such as boron, chromium, copper, and nickel. These enhance the hardenability effect of molybdenum by metallurgical synergies. Besides, chromium adds to the corrosion resistance under aggressive operating conditions while nickel enhances toughness especially at low operating temperatures. Vanadium provides efficient secondary hardening by precipitation during the tempering treatment.
Pressure vessel steels
Low-alloy chromium-molybdenum steels (1-3 % Cr and 0.5- 1 % Mo) are standardly selected for the fabrication of heat exchangers and process reactors. CrMo steels have been used for more than 50 years in chemical and petrochemical plants. With the evolution of process parameters these steel grades have been developed towards enhanced properties accordingly:
- Conventional CrMo steels: ASTM A387 Gr.11 Cl 1 and 2 or Gr. 22 Cl. 2 or 13CrMo4-5 or 10CrMo9-10 acc. EN 10028 part2
- Enhanced CrMo steels: quenched and tempered steels with increased mechanical properties, e.g. ASTM A 542A/B 3/4/4a or 12CrMo9-10 acc. EN 10028 part 2
- New generation of CrMo steels: Vanadium modified CrMo-steels, e.g. ASTM A542 D4a or 13CrMoV9-10 acc. to EN 10028 part 2.
Vessels for elevated high-pressure hydrogen-resistance specifications, as in the case of hydrotreaters, hydrodesulfurizers and hydrocrackers, for example, are constructed from plate with sophisticated specifications. The Nelson diagram defines the limits of operating and hydrogen partial pressure for the different steel grades. Petrochemical process reactors are designed to cope safely with the longest possible campaign times under the simultaneous exposure to high pressures, high temperatures and attack by pressurized hydrogen. Important mechanical and technological data is strength at room temperature, at elevated temperature and in the creep range as well as the impact energy. It should be noted that it is not only chemical composition and plate thickness, which influence materials properties. Heat-treatment conditions (normalizing, tempering, water quenching and tempering, and stress-relieving annealing) and, where appropriate, hot forming during further processing and working all play a decisive role. In addition, the material properties in the long-term service can change also after manufacturing of the pressure vessels.
Abrasion resistant steels
Machines and equipment for industry, mining, agriculture, and other constructions often apply heavy plates, which have to guarantee good wear resistance. The most typical application is for machines to excavate raw materials such as coal, ores, minerals etc. These steels comprise as characteristic alloying elements manganese, chromium, molybdenum, and nickel and are based on a carbon levels up to 0.40%. Consequently, they attain high hardness values of 400 to 600 HB. Typical plate thicknesses range up to 100 mm.
The characteristic wear type in most applications is ploughing leading to abrasive wear. Thereby usually the plate surface is being scratched when exposed to an abrasive and hard material such as mineral particles or other metals. High hardness of the steel is one important feature for good wear resistance. Additionally, also a higher toughness of the material improves the wear resistance and thus reduces material loss.
Welding of structural steels
Most often structural steels are subjected to intensive welding operations during construction, maintenance, and repair. The most important criterion to judge weldability of a steel alloy is the carbon equivalent. Different definitions of this parameter exist. The CEV and the CET value criteria are commonly used for structural steels:
CEV = C + Mn/6 + (Cr+Mo+V)/5 + (Cu+Ni)/15
CET = C + (Mn+Mo)/10 + (Cr+Cu)/20 + Ni/40
The higher the carbon equivalent, the worse is the steel’s weldability. It is particularly effective to reduce the carbon content in a steel to improve the weldability. This is simultaneously beneficial for toughness. The potential loss of strength has to be regained by other alloying elements and thermomechanical (TMCP) treatment during production of the plate material. In order to comply with a maximum specified carbon equivalent, the alloy composition has to be optimized according to the weighting factors in the CE criterion. TMCP rolling in combination with accelerated cooling always allows a lower carbon equivalent in comparison to equally strong QT-steels. However, the thickness range for TM steels is becomes limited as the strength increases.
The cooling time from 800 to 500°C after welding (ΔT8/5) is a decisive criterion with regard to the properties in the heat affected zone. While the carbon content controls the plateau hardness of the martensite at very short cooling time, the CE determines at which cooling time martensite formation occurs. With increasing CE the critical ΔT8/5 is shifted to longer times and tends to be in the typical working range of SMAW and MAG welding processes used for on-site welding of plate sections. Grades with a comparably high CE demand special precautions for welding including pre-heating of the weld zone. The process window is becoming narrower as the CE rises. Welding with too high heat input is detrimental to strength and toughness whereas a too small heat input would result in excessive hardness and enhanced cold cracking susceptibility in the HAZ. The welding consumables that produce tensile properties overmatching the ones of 690 or 890 MPa QT-steel demand a relatively high alloying content. A combination of 1-2.5% Ni, 0.5-1.5% Cr and about 0.5% Mo is typical. Due to this chemical composition and its as cast microstructure, weld metals for such ultra-high strength steels are often more susceptible to hydrogen induced cold cracking than the HAZ of the parent material. In order to avoid cracking, preheat and interpass temperature must then be adapted to the weld metal. Steel with reduced carbon equivalent do not allow dropping these the precautions in welding. Consequently, high strength parent material with reduced carbon equivalent, as achieved by TMCP–rolling, need essentially the same precautions as the higher alloyed QT steel.

HAZ hardening of various high strength plate grades in function of cooling rate after welding

Welding process windows for different high strength plate grades