Line pipe steels
Advantages: high strength, potential for weight and material savings
Typical applications: large diameter pipe for oil and gas transmission
Effects of molybdenum: discourages pearlite formation after finish rolling, promotes good combination of strength and low-temperature toughness
Since well over fifty years, the most economic and efficient way to transport natural gas and crude oil across long distances is through large diameter steel pipelines. These large pipelines span diameters from 20” to 56” (51 cm to 142 cm) but typically range between 24” to 48” (61 cm to 122 cm).
As global energy needs increase and new gas fields are found in increasingly harsh and remote locations, requirements for greater transmission capacity and increased safety of pipelines drive final design specifications along with cost. Fast developing economies like, China, Brazil and India have further spurred the demand for pipe.
The demand for large diameter pipe has outgrown the available supply from traditional manufacturing routes using heavy plate in an UOE (U-forming O-forming E-xpansion) pipe, resulting in a processing bottleneck. Consequently, the relevance of large diameter, heavy gauge spiral pipe produced from hot strip has grown tremendously.
The use of High Strength Low Alloy Steels (HSLA) was established in the 1970s when the introduction of the thermomechanical rolling process, coupled with microalloying with niobium (Nb), vanadium (V) and/or titanium (Ti), permitted higher strength steels to be made without additional expensive heat treatment processes. Typically, these early HSLA line pipe steels relied on pearlite-ferrite microstructures to make pipe steel grades up to X65 (min. yield strength of 65 ksi).
Over time, calls for higher strength pipelines led to extensive research in the 1970s and early 1980s for developing strengths of X70 or higher using low carbon steel designs, many employing a molybdenum-niobium alloying concept. However, with the introduction of new process technology such as accelerated cooling, it became possible to develop higher strengths with much leaner alloy designs.
Nevertheless, whenever rolling mills are not capable of applying the required cooling rates on the run-out-table, or do not even have the necessary accelerated cooling equipment, the only practical solution is using selected additions of alloying elements to develop the desired steel properties. With X70 becoming the workhorse of modern pipeline projects and the increasing popularity of spiral line pipe, the demand for cost-effective heavy gauge plate and hot-rolled coil produced in both Steckel mills, or conventional hot-strip mills, have grown significantly over the past several years.
More recently, the first large-scale projects using X80-grade material for long-distance large diameter pipe were realized in China. Many of the mills supplying into these projects use alloying concepts involving molybdenum additions based on metallurgical developments made during the 1970s. Molybdenum-based alloy designs have also proved their value for lighter gauged medium diameter pipes. Here, the driving force is efficient pipe installation and high operational reliability.
Since they came to market, the operating pressure of gas transmission pipelines has increased from 10 to 120 bars. With the development of the grade X120, the operating pressure may potentially further increase to 150 bars. Increasing the pressure requires the use of thicker walled steel pipe and/or higher strengths. As the total materials costs can account for over 30% of the overall pipeline costs for an onshore project, reducing the amount of steel used through higher strengths can amount to significant cost savings.
The figure below indicates that when the minimum yield strength of the pipe is doubled, around half of the steel is required, depending on the size and operating conditions. For instance, the Ruhrgas project in the 1990s demonstrated that upgrading from X70 to X80 pipe steel grade could save 20,000 tonnes or 12% of steel with the same transmission capacity of the pipeline.
Alternatively, upgrading the material strength allows increasing pipe diameter and operating pressure resulting in a higher throughput. Importantly, savings also extend to the associated pipe welding costs, transportation, and handling. In some long-distance projects, pipes must be transported to remote areas where lighter weights reduce logistical complications.
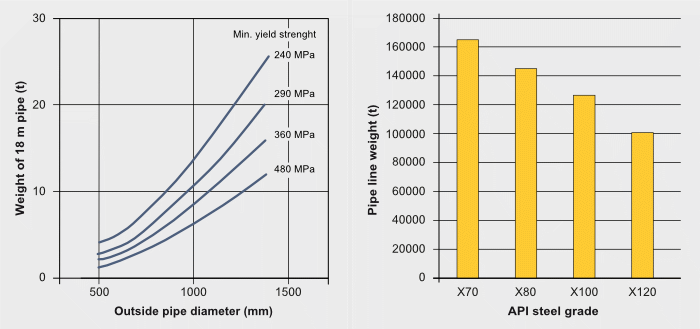
Weight to diameter ratio in pipes at constant pressure as a function of the strength; weight saving potential by upgrading strength, example of the Ruhrgas project.
Reference: High-strength Large-diameter Pipe for Long-distance High Pressure Gas Pipelines
M. K. Gräf, H.-G. Hillenbrand, C. J. Heckmann, K. A. Niederhoff
Proceedings of The Thirteenth (2003) International Offshore and Polar Engineering Conference Honolulu, Hawaii, USA, May 25–30, 2003
The drive towards higher strength pipes and increased operating pressures also means that modern pipes must have excellent resistance to both brittle and ductile fracture propagation. Increasing the hoop stress in the pipe translates to higher impact energy requirements. For today’s requirements, the Charpy 50% FATT (Fracture Appearance Transition Temperature) and the Battelle DWTT (Drop Weight Tear Test) at 85% FATT are often specified at -20°C and -15°C, respectively, and at even lower temperatures in some project specific cases. Specifiers generally emphasize the desired level of toughness for resistance to ductile fracture propagation, which translates to an effective minimum Charpy upper shelf energy.
To deliver such high strengths and excellent toughness properties, the microstructure of the steel pipe must change from a traditional ferrite-pearlite structure towards a lower carbon ferrite-acicular ferrite or low carbon bainite microstructure. One of molybdenum’s key metallurgical effects is to retard or fully avoid the formation of pearlite during the transformation from austenite after finish rolling.

Comparison of low-temperature Charpy toughness between industrially produced X70 alloy concepts.
The variety of pipe steel production routes is shown in the figure above, which indicates the key processing techniques, steel chemistry designs, and typical microstructures. For good weldability and high toughness, the carbon content in modern pipe steel is preferably sub-peritectic, i.e., below 0.10 wt%. The specified strength of the plate or strip steel can be achieved by various strengthening mechanisms such as:
- Grain refinement (by microalloying and thermomechanical rolling)
- Solid solution hardening (by addition of bulk alloying elements)
- Introduction of dislocation substructures (by accelerated cooling and Mo alloying)
- Phase transformation strengthening (by accelerated cooling and Mo alloying)
- Precipitation hardening (by microalloying, assisted by Mo alloying)
Grain refinement obtained through controlled rolling in combination with microalloying is the cornerstone of all modern pipe steel production concepts. This is because grain refinement is the only strengthening mechanism that also provides better toughness. All other strengthening mechanisms reduce toughness. Regarding the complete property profile of the final pipe steel, the combination of grain refinement, dislocation substructures, and phase transformation strengthening has demonstrated the best potential.
The first molybdenum-based pipe steel alloys for X70 strength level were developed in the 1970s. The table below details the evolution of pipe steel alloying concepts in plate rolling over three decades. The introduction of accelerated cooling after thermomechanical rolling in the 1980s allowed the production of pipe steel with a partially bainitic microstructure, even without molybdenum alloying.
Increasing demands by the oil and gas industry for strength levels above X70, capable of withstanding lower operating temperatures, heavy wall gauges and strain-based design resulted in a reintroduction of molybdenum alloying. Such steels require an increased level of manganese and additional alloying with chromium, nickel, and/or copper.
Evolution of alloy designs for high-strength pipe grades produced by plate mill rolling (FP: ferrite-pearlite, PR: pearlite reduced ferrite, AF: acicular ferrite)
Year
|
Grade
|
Process
|
%C
|
%Mn
|
%Mo
|
%Ni
|
%Cu
|
%V
|
%Nb
|
%Ti
|
%B
|
1970
|
X70 FP
|
TM
|
0.10
|
1.50
|
|
|
|
0.05
|
0.04
|
|
|
1975
|
X70 PR
|
0.10
|
1.40
|
0.20
|
|
|
|
0.05
|
|
|
|
1975
|
X70 AF
|
0.06
|
1.85
|
0.30
|
|
|
|
0.06
|
|
|
|
1985
|
X70
|
TM + acc
|
0.07
|
1.60
|
|
|
|
|
0.04
|
|
|
1990
|
X80
|
0.07
|
1.85
|
0.15
|
|
|
|
0.05
|
0.02
|
|
|
1995
|
X100
|
TM + acc (DQ)
|
0.07
|
1.90
|
0.30
|
0.25
|
0.25
|
|
0.05
|
0.02
|
|
2002
|
X70 heavy wall
|
TM + acc
|
0.06
|
1.70
|
|
0.20
|
0.20
|
|
0.05
|
0.02
|
|
0.06
|
1.70
|
0.15
|
|
|
|
0.05
|
0.02
|
|
|
|
|
0.05
|
1.70
|
|
|
|
|
0.08
|
0.02
|
|
|
|
|
2002
|
X80 arctic
|
TM + acc
|
0.06
|
1.90
|
0.20
|
0.20
|
|
|
0.05
|
0.02
|
|
2003
|
X120
|
TM + acc (DQ)
|
0.05
|
1.95
|
0.50
|
1.50
|
0.50
|
|
0.05
|
0.02
|
0.002
|
2006
|
X80 high strain
|
TM + acc
|
0.06
|
1.75
|
0.20
|
0.25
|
0.30
|
|
0.02
|
0.01
|
|
Increasing demand for large diameter pipe, combined with limited plate rolling capacity promotes the use of spiral pipe for long-distance pipeline projects. Spiral pipe making is based on hot-rolled strip, which was traditionally applied for thinner gauges. Appropriate modifications in strip mills nowadays allow the production of up to one-inch heavy strip for pipe.
Several significant differences in the time-temperature-deformation schedule of strip compared to plate mills necessitate different alloying concepts for obtaining a specified pipe grade. The accelerated cooling of heavy gage hot strip is less intense compared to plate, as the strip passes the cooling section with high speed.
Molybdenum alloying is particularly efficient, relative to other elements, in retarding ferrite or pearlite formation under such cooling conditions. Solute molybdenum promotes the transformation into an acicular ferritic (AF) microstructure, which offers an attractive combination of strength and toughness. In several recent pipe projects, the specified microstructure was AF, and consequently molybdenum alloying was highly relevant, especially for larger wall gauge pipes.